Anno Accademico 2021-2022
Vol. 66, n° 3, Luglio - Settembre 2022
Settimana per la Cultura
12 aprile 2022
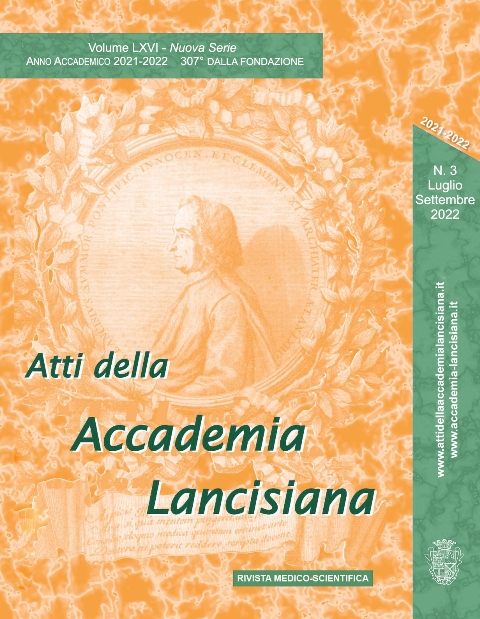
Settimana per la Cultura
12 aprile 2022
Versione PDF dell'articolo: Download
Diffuse Intrinsic Pontine Glioma (DIPG) is a highly aggressive paediatric brainstem tumour, with a yearly incidence of 2.32 per 1.000.000, no gender predilection and a median age at diagnosis of 6-9 years. Less than 10% of DIPG patients survive for 2 years, with a median overall survival of 9-12 months. Due to the anatomical location and the diffusely infiltrative nature of the tumour, current treatment options are limited and progress towards clinical improvement has been stationary throughout the last four decades. Until recently, a paucity of tissue samples to study led to a lack of understanding of the underlying molecular components of these tumours. Focal radiation therapy is the current standard-of-care for children with newly diagnosed DIPG. Nevertheless, this treatment provides significant but only temporary efficiency delaying tumour progression (Donaldson et al., 2006). Often alongside the radiotherapy, some forms of chemotherapy are provided in the context of a clinical trial to improve outcome, but no chemotherapeutic agent has demonstrated significant efficacy.
Patients with DIPG can present with a wide variety and usually with an acute onset of neurological symptoms and signs reflecting the anatomic localization and the extent of the lesion. Commonly reported symptoms include abnormal or limited eye movements, diplopia, facial asymmetry, clumsiness, disturbance of gait and loss of balance, and weakness. Symptoms are usually of rapid onset with short duration (1-2 months) prior to diagnosis. Cranial nerves VI and VII are the most affected and specific dysfunction of these is characteristic of DIPG.
The diagnosis of DIPG is based on typical clinical presentation and characteristic neuroimaging findings. MRI is the imaging modality of choice for diagnosis. On MRI, DIPGs demonstrate T1-hypointensity and hyperintensity on T2- weighted images, generally without contrast enhancement, and frequently appear relatively homogeneous on fluid attenuated inversion recovery (FLAIR) sequences. Contrast- enhancement is variable, but these lesions frequently do not significantly enhance at diagnosis. On imaging, the tumour core is centred in the pons and diffusely involves most of its axial diameter (Fig. 1).
![]() |
|
For decades, the role of neurosurgery in DIPG has been limited to evaluation and treatment of obstructive hydrocephalus from DIPG and rare biopsies for cases of uncertain diagnosis. However, with the improvements in the biological, molecular and genetic understanding of this disease, tissue acquisition, and therefore surgical biopsy, has developed an increasingly critical role. Developments in identification of potential treatment targets for these lesions, together with the increased safety of stereotactic brain stem biopsies, have helped move the field forward (Hamisch et al., 2017; Williams et al., 2020). Improvements in the safety and feasibility of brain stem biopsy have occurred coincident with improved understanding of DIPG biology. Stereotactic brainstem biopsy in all cases of newly diagnosed DIPG represents a means to better understand the disease and to characterize individual tumours based on molecular pathology in order to direct individualized treatment.
![]() |
|
Recent studies have shed light on the molecular makeup of diffuse intrinsic pontine gliomas and identified the H3K27M mutation in nearly 80% of DIPGs, leading to the 2016 WHO classification of diffuse midline glioma H3K27M-mutant, a grade IV brain stem tumour. The histone mutation H3K27M results in the substitution of lysine with methionine at position 27 in the isoforms H3.1 and H3.3, encoded by genes HIST1H3B and H3F3A respectively (Wu et al., 2012). This substitution occurs at a critical position within the N-terminal histone tail submitted to regulatory post-translational modification associated with transcriptional repression (K27). This mutation leads to the loss of histone trimethylation, via inhibition of polycomb repressive complex 2 (PRC2), with global hypomethylation of the lysine at position 27 of the H3 protein (H3K27), ultimately producing epigenetic silencing and dysregulation of gene expression (Bender et al., 2013).
There are subtle differences between the histone mutations in H3.1 and H3.3, particularly regarding survival, phenotype, and clinical outcomes (Castel et al., 2015; Khuong-Quang et al., 2012). K27M-H3.1 mutations are specific to the pons with a significantly longer survival (median 15.0 months), whereas K27M-H3.3 are found alongside midline structures i.e. pons, thalamus and spinal cord (Mackay et al., 2017) (Fig. 2). Patients who harbor the K27M- H3.3 mutation have worse overall survival in comparison with other H3 wild-type cases (Khuong-Quang et al., 2012) (Fig. 3).
![]() |
|
In addition, clonal missense ACVR1 mutations were found exclusively in DIPGs (32%) and co-segregate with H3.1 (Wu et al., 2014). ACVR1 gene encodes a bone morphogenetic protein (BMP) type I receptor and ACVR1 mutations in DIPG activate BMP signaling. ACVR1 mutations cause a constitutive ligand-independent activation of the TGFβ/BMP signaling pathway, resulting in increased levels of SMAD phosphorylation, as well as overexpression of downstream targets ID1 and ID2 (Buczkowicz et al., 2014; Wu et al., 2014). All the ACVR1 residues affected by mutation in DIPGs clustered around either the inhibitory glycine-serine-rich (GS) domain or the ATP-binding pocket of the kinase domain and would be expected to shift the kinase to an active conformation (Wu et al., 2014) (Fig. 4).
![]() |
|
The standard of care of newly diagnosed DIPG patients is focal radiation therapy (RT), using a 1 cm margin to cover microscopic disease, to a total dose of 54 Gy administered over 6 weeks, usually in daily 1.8-2 Gy fractions. Glucocorticoids are frequently prescribed at the time of diagnosis to reduce and control edema associated with the tumour and radiation treatment. While high-dose steroids offer early relief, their adverse effect profile, including impaired sleep, wound healing, behavior, and endocrine and metabolic functional effects, limits their long-term utility (Curtis et al., 2006). Although large numbers of therapeutic approaches have been tested, no significant progress has been made in treating these high-grade gliomas. Therefore, the identification of new specific targeted therapies is of great importance for innovative and effective treatments.
In this context, the aim of my thesis was to evaluate molecular aspects and heterogeneity, in a cohort of DIPGs patients, and to use this information for the choice of a personalized targeted therapy. The goal of this study was to assess the overall survival and progression-free survival of children with DIPGs receiving a personalized approach with molecularly targeted agents based on tumour molecular profiling. The study retrospectively identified a cohort of 25 patients treated at Bambino Gesù Children’s Hospital in Rome from 2014 to 2019, with a radiologic diagnosis of DIPG. Treatment at diagnosis was focal radiotherapy (54 Gy administered in daily 1.8 Gy fractions 5 days a week) associated with systemic chemo-immunotherapy as by institutional guidelines based on Massimino et al (2014) indications. Second line treatment included heterogeneous patients-adapted administration regimens.
In order to design personalized treatments, potentially targetable alterations were screened using a combination of immunohistochemistry, Sanger sequencing and Next generation sequencing (NGS). The most frequently targetable mutations were mTOR/pmTOR pathway mutations known to increase sensitivity to mTOR inhibitors such as everolimus and activating ACVR1 mutations. Other potentially actionable alterations included BRAF mutation and a mutation of PDGFRA known to increase sensitivity to kinase inhibitors such as pazopanib.
Guided by comprehensive molecular profiling on tumour tissue, 9/25 patients were subjected to personalized treatment at primary diagnosis (1/9) and at disease progression and received backbone therapy including focal irradiation. Personalized treatments included inhibition of the mTOR pathway, immunotherapy, retinoic receptor agonist, receptor tyrosine kinase inhibition.
Median overall survival (OS) of the whole cohort was 17.6 months whereas median progression free survival (PFS) was 7.63 months (Fig. 5).
![]() |
![]() |
Fig. 5. PFS and OS curves for the whole cohort. |
![]() |
|
We found a significant association between TP53 staining and OS. Patients with positive TP53 staining (> 50%) showed a significant (p= 0.022) shorter overall survival (HR=3.15 CI:1.123-8.834) as shown in Figure 6. Median survival for patients with positive immunostaining of TP53 was 13.2 months versus 20.2 months median survival for patients with p53 < 50%.
Similarly, we confirmed in our cohort that H3F3A mutation confers worst survival. Patients harboring H3F3A mutations had a median OS of 14.2 months versus a median of 24.5 months for patients with mutations in HIST1H3B (HR: 3.41 CI:1.231-9.443, p= 0.01) (Fig. 7).
Then, we evaluated the potential impact of personalized treatment approaches on survival compared to the control group. Kaplan-Meier survival analysis revealed significantly better overall survival for patients treated with targeted therapies versus control group with a median survival of 20.05 vs 14.3 months. The difference in survival was significant (p= 0.03) with an HR= 0.345 (CI:0.1236-0.9667) (Fig. 8).
![]() |
![]() |
|
|
Major limitations of the study were reduced patient number, non-prospective design of the study and heterogeneity of population in terms of molecular biology and treatments.
Overall, this study details the institutional experience at Bambino Gesù Children’s Hospital performing personalized targeted approaches in paediatric neuro-oncology patients. We highlighted the feasibility of genomic profiling in both the primary and recurrent disease setting. Moreover, we presented the significant impact this testing is having on the identification of potential actionable molecular alterations and the improved patient outcome through a personalized approach in H3K27M gliomas.
Based on the results described in our study, we propose to consider biopsy in all patients with DIPG at diagnosis, in order to detect potential molecular drivers alterations that can be targetable and, subsequently, to add personalized agents in first-line treatment in DIPG patients along with the backbone treatment. We envision that this testing will serve as the basis for future clinical trials of personalized targeted therapy, leading to improved outcomes for children with brain tumours.
Nevertheless, large series and future clinical trials are needed to validate these interesting data.
Sintesi della Tesi di Laurea discussa il 28 giugno 2021
Facoltà di Farmacia e Medicina, Dipartimento di Medicina Sperimentale, Corso di Laurea in Medicina e Chirurgia, “Sapienza” Università di Roma
Relatore: Prof.ssa Elisabetta Ferretti, Facoltà di Farmacia e Medicina, Dipartimento di Medicina Sperimentale, “Sapienza” Università di Roma
Correlatore: Dott.ssa Angela Mastronuzzi, Responsabile Unità di Neuro-Oncologia Ospedale Pediatrico Bambino Gesù, Roma
BIBLIOGRAFIA
Bender S, Tang Y, Lindroth AM, et al. Reduced H3K27me3 and DNA hypomethylation are major drivers of gene expression in K27M mutant pediatric high-grade gliomas. Cancer Cell 2013; 24: 660-72.
Buczkowicz P, Hoeman C, Rakopoulos P, et al. Genomic analysis of diffuse intrinsic pontine gliomas identifies three molecular subgroups and recurrent activating ACVR1 mutations. Nat Genet 2014; 46: 451.
Castel D, Philippe C, Calmon R, et al. Histone H3F3A and HIST1H3B K27M mutations define two subgroups of diffuse intrinsic pontine gliomas with different prognosis and phenotypes. Acta Neuropathol 2015; 130: 815-27.
Curtis JR, Westfall AO, Allison J, et al. Population-based assessment of adverse events associated with long-term glucocorticoid use. Arthritis Rheum 2006; 55: 420-6.
Donaldson SS, Laningham F, Fisher PG. Advances toward an understanding of brainstem gliomas. J Clin Oncol 2006; 24: 1266-72.
Hamisch C, Kickingereder P, Fischer M, Simon T, Ruge MI. Update on the diagnostic value and safety of stereotactic biopsy for pediatric brainstem tumors: a systematic review and meta- analysis of 735 cases. J Neurosurg Pediatr 2017; 20: 261-8.
Khuong-Quang DA, Buczkowicz P, Rakopoulos P, et al. (2012). K27M mutation in histone H3.3 defines clinically and biologically distinct subgroups of pediatric diffuse intrinsic pontine gliomas. Acta Neuropathol 2012; 124: 439-47.
Mackay A, Burford A, Carvalho D, et al. Integrated Molecular Meta-Analysis of 1,000 Pediatric High-Grade and Diffuse Intrinsic Pontine Glioma. Cancer Cell 2017; 32: 520-37e5.
Massimino M, Bode U, Biassoni V, Fleischhack G. Nimotuzumab for pediatric diffuse intrinsic pontine gliomas. Expert Opin Biol Ther 2011; 11: 247-56.
Warren KE. Diffuse intrinsic pontine glioma: poised for progress. Front Oncol 2012; 2: 205.
Warren KE, Killian K, Suuriniemi M, Wang Y, Quezado M, Meltzer PS. Genomic aberrations in pediatric diffuse intrinsic pontine gliomas. Neuro Oncol 2012; 14: 326-32.
Williams JR, Young CC, Vitanza NA, et al. Progress in diffuse intrinsic pontine glioma: advocating for stereotactic biopsy in the standard of care. Neurosurg Focus 2020; 48: E4.
Wu G, Broniscer A, McEachron TA, et al. Somatic histone H3 alterations in pediatric diffuse intrinsic pontine gliomas and non-brainstem glioblastomas. Nat Genet 2012; 44: 251-3.
Wu G, Diaz AK, Paugh BS, et al. The genomic landscape of diffuse intrinsic pontine glioma and pediatric non-brainstem high-grade glioma. Nat Genet 2014; 46: 444-50.